Black Body Radiation
A black body is an object in physics that takes in all energy, no matter what kind it is. It doesn't reflect any radiation, so it looks black to our eyes. However, black bodies can also give off radiation, which is called black body radiation. This radiation is released perfectly without any loss of energy. When black bodies are in balance, their temperature is related to their emission properties. This was first studied by Gustav Kirchhoff, a scientist.
The reason why perfect absorbers are also perfect emitters is because the two processes are opposite to each other. Electromagnetic energy is absorbed and emitted by photons. This is the process that takes place in black bodies. Black body radiation is an essential concept in physics that helps us understand the behavior of energy.
The definition of black body radiation
When a black body is in equilibrium, the amount of energy entering a region of the object equals the energy leaving the same region. The radiation emitted by a black body is known as black body radiation, which has a continuous spectrum known as the black body spectrum, whose pattern is very well known (see the image below for examples).
Why does classical mechanics fail to explain the blackbody spectrum?
Classical mechanics couldn't explain the black body spectrum because the models suggested that particles could vibrate at higher energies without any limit as the intensity increased. This would lead to a spectrum with more intense vibrations at higher frequencies. However, the black body spectrum showed that intensities decreased as the wavelength approached zero. The red, green, and blue curves in the image below represent different temperatures, with the peaks of the emission intensity corresponding to different frequencies depending on the temperature.
To solve this disagreement between theory and experiments, Max Planck proposed an idea at the end of the nineteenth century. He suggested that energy wasn't shared between particles on arbitrary continuous values, but only in fixed amounts that were multiples of a number "h". For high frequencies of light, kinetic energy is calculated as h·f (where f is frequency). It can't be translated into thermal energy at these frequencies because it would mean infinite temperatures. The constituents of the black body gradually move towards a region where they have no temperature, as seen in the left side of the image. The right side of the image was understood without quantum physics because, for very low frequencies, the equivalent thermal energy is also very low. The understanding of high-frequency regimes led to the prediction of the existence of photons, which carry discrete pieces of energy measured by the constant h.
Characteristics of the black body spectrum
The most intense radiation emitted by an object has a wavelength λ that's inversely proportional to the temperature. This means that higher temperatures emit radiation at shorter wavelengths, resulting in a shift towards the blue spectrum. The spectrum of radiation has some specific characteristics: it's continuous, and the spectrum peak moves towards shorter wavelengths as the temperature increases. At 0, the spectrum is 0 due to the quantisation of energy, and the spectrum values at larger wavelengths are smaller.
Black body radiation can be used to model astronomical objects, which is why it's so important in physics, astrophysics, and astronomy.
Black body radiation colour chart
Temperature, wavelength emission, and perceived color are related quantities for a black body. A color chart can show the dependence of black body temperature on color. In astronomy, we can estimate the temperatures of astronomical objects by characterizing their emission properties, as shown in the image below.
The colors observed for any object are the result of the light (photons) emitted or reflected by the object. Photons have different wavelengths depending on their energy: low energy photons are perceived as red, and high energy photons are perceived as blue-violet. The difference in color perception is due to cone cells, which are special photoreceptor cells that can convert incident light into an electrical signal delivered to the brain. Cone cells are divided into three types that are sensitive to a range of different wavelengths: short wavelengths like blue and purple, medium wavelengths like green and yellow, and long wavelengths like orange and red.
Stars and black body radiation
can be modeled as black bodies, and their surface temperature and wavelength emission are related. Because of this relationship, we can differentiate which stars have a higher temperature than others by the radiation they emit and the observed color. See the table below for some examples (where K stands for Kelvin and nm for nanometers):
Stars do not exhibit the same color throughout their lifetimes, and the variation in color can tell us a lot about the temperature and processes happening inside.
One example is the specific case of a yellow star, like our Sun, which will become a red giant.
A star exists due to a process of nuclear fusion that occurs inside it. This process primarily fuses hydrogen and helium, creating heavier elements. During fusion, the core of a star is in equilibrium, with gravity trying to compress it and fusion trying to expand it.

As the hydrogen is exhausted, the star score starts to compress due to gravity as the force balance is broken and the compression heats the nucleus. As a result, the star’s outer layers heat up, and the fusion process happens in the outer layers.
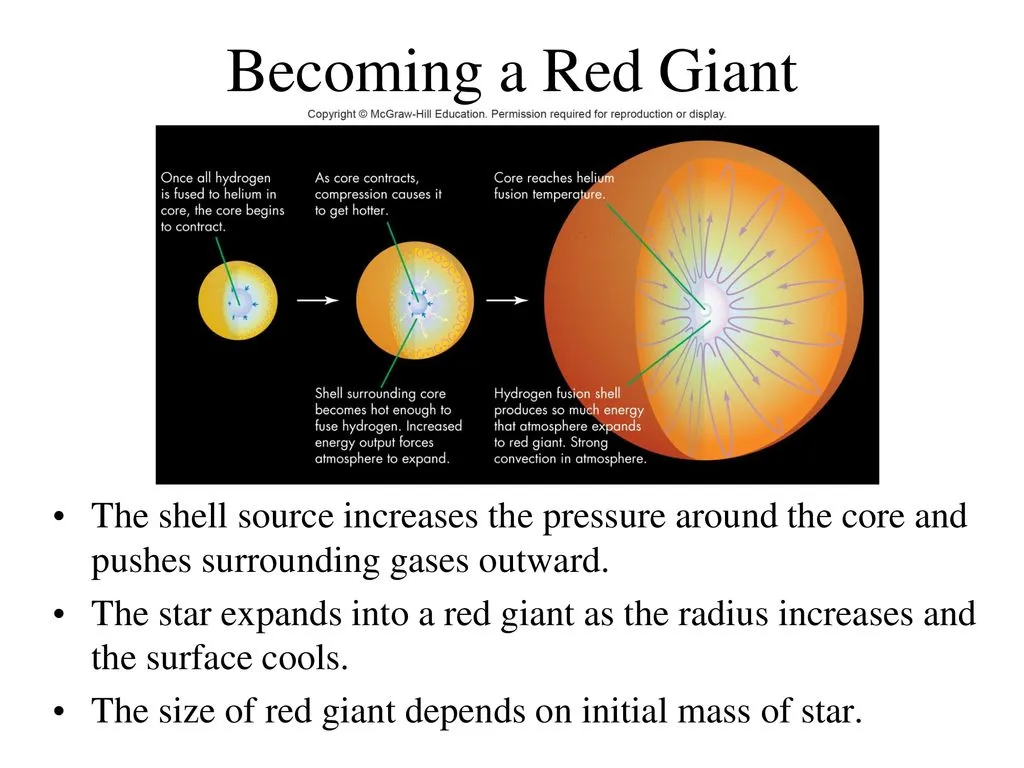
As the process expands the star's outer surface, the surface cools down. During this process, the star becomes a red giant. As a result, the star's color changes from yellow to red, and its emission shifts to larger wavelengths (lower surface temperatures).
If you go outside on a clear night in some parts of the Northern Hemisphere, you may be able to see two stars: Betelgeuse and Rigel. In the image below, the upper left red star is Betelgeuse. This star is a red supergiant, and as such, it must have a peak emission on the red spectrum. The star below Betelgeuse to the right is Rigel, and its blue color indicates that its spectral peak must be around a blue-purple color.
The emission peak of Rigel is measured at around 145nm, while the emission peak of Betelgeuse is measured at around 855nm. If you look at the table of color spectra, you can see that the color of Betelgeuse corresponds to a larger wavelength (red colors) and Rigel to a shorter wavelength (purple-blue colors). Due to the law that connects the energy of photons with the frequency, we know that the photons emitted by Rigel have more energy than the photons emitted by Betelgeuse.
Application of black body radiation to blackholes
Black holes are astronomical objects that can be modeled as black bodies, in this case as perfect absorbers that do not let any radiation escape. Black holes are still under heavy research, but they are expected to emit some kind of radiation as perfect absorbers.
A suggested mechanism proposed by the British scientist Stephen Hawking – known as Hawking radiation – takes the form of a black body radiation curve. However, no definitive answers have been found for these phenomena.
To summarize, a black body is a perfect emitter and absorber of radiation. In a black body, the radiation emission depends on its temperature. Because of the temperature-emission-frequency relationship, the emission peak gives us the body's temperature. Stars, as emitting bodies, can be modeled as black bodies. Because of this, the color of the star can tell us about its temperature. Red stars will be colder, and blue stars will be hotter. Black holes are perfect black bodies, able to absorb all radiation. Several mechanisms have been predicted and theorized by which they can emit radiation. One of them is the Hawking radiation.
Black Body Radiation
What is black body radiation?
Black body radiation is the radiation emitted by a perfect absorber and emitter(a black body).
Why does classical theory fail to explain black body radiation?
Classical theory predicted that the intensity of emission would increase asfrequencies became higher. However, it did not take into account quantumeffects that become relevant at very high frequencies and lead to a drop in theintensity of emission.
How does black body radiation prove the particle nature of light?
As the explanation given by Max Planck required light (radiation) to have discretevalues, the light had to be emitted in small packages or particles known asphotons. This is how black body radiation proves the particle nature of light.
What is the black body radiation spectrum?
When a black body is in equilibrium, the amount of energy entering a region ofthe object equals the energy leaving the same region. The radiation emitted bya black body is known as black body radiation, which has a continuous spectrumknown as the black body spectrum, whose pattern is very well known.
Who discovered black body radiation?
Black body radiation was predicted by Gustav R. Kirchhoff. However, it was MaxPlanck who was able to describe the behaviour of black body radiation.