Understanding NMR
As a chemist, you spend your days experimenting with different substances in the lab. Sometimes, you might create a new compound, but how can you be sure that it's never been discovered before? That's where NMR spectroscopy comes in. NMR stands for nuclear magnetic resonance, and it's a technique used by scientists to figure out the structure of different molecules.
NMR spectroscopy is used in many fields, from analysing proteins to MRI scans at hospitals. In this article, we'll explore how NMR works and explain concepts like spin and resonance frequency. We'll also discuss two types of NMR: carbon-13 NMR and proton NMR. By understanding NMR spectroscopy, you can take your chemistry experiments to the next level and discover new compounds with confidence.
What is NMR?
To understand what NMR actually is, let’s look at its name a little more closely: nuclear magnetic resonance spectroscopy. 'Nuclear' refers to nuclei, as you can probably guess. Remember that a nucleus is a dense mass found in the centre of an atom, containing protons and neutrons. 'Resonance' is a type of energy emitted by molecules. If we put that all together, we can work out that NMR measures the resonance energy of nuclei using magnetic fields.
How does NMR work?
Let’s take a look at how exactly NMR works. To do this, we need to investigate several different ideas. Nuclear spin.Parallel and antiparallel states.Resonance.
Nuclear spin
Cast your mind back to the start of your days learning chemistry, to the model of the atom. You know that an atom contains protons and neutrons in a central nucleus, surrounded by orbiting electrons. Protons and neutrons are collectively called nucleons. An atom. The red and yellow particles represent protons and neutrons, collectively known as nucleons.

You might also remember that electrons have a property called spin. This takes two different directions: up and down. When electrons pair up in orbitals, one electron has an up spin and the other has a down spin. Nucleons have spin too. Each proton or neutron has a spin of ½. If there is an even number of either protons or neutrons, their spins cancel out and there is no spin at all. But if there is an odd number, we are left with a net spin of ½. If there is an odd number of both protons and neutrons, we are left with a net spin of ½ + ½ = 1.
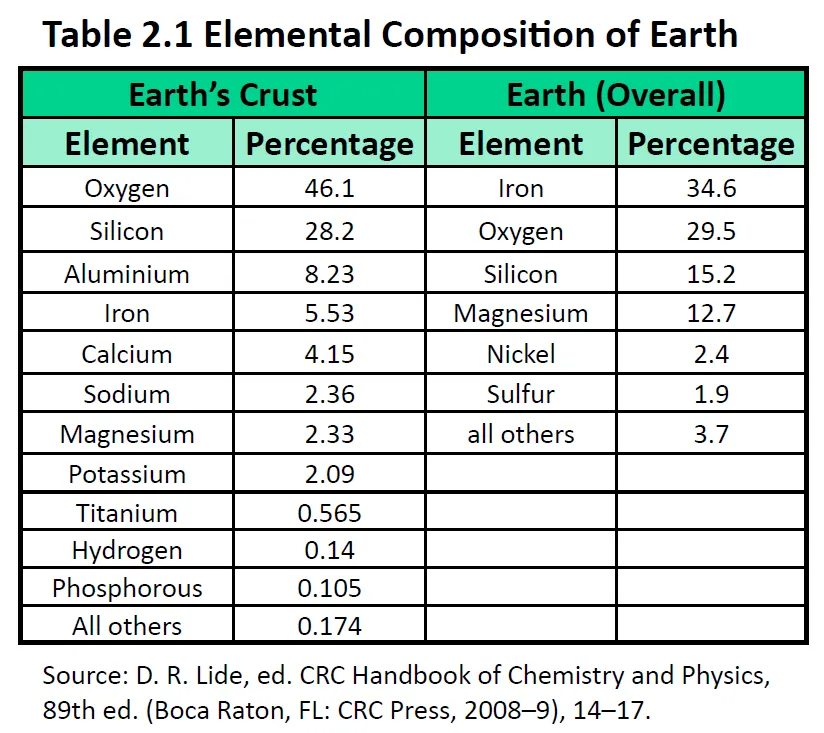
Helium, shown on the left, has an even number of both protons and neutrons. It has no overall spin.
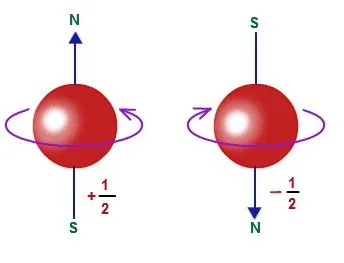
Parallel and antiparallel states
You may have heard that nuclei can have spin, but what does that actually mean? Well, spin makes nuclei act like tiny magnets when they're exposed to magnetic waves. Think of it like a compass needle. When it's left alone, it lines up with Earth's magnetic field and points to the north pole. If you turn it to point south, it will quickly spin back around to its original position because pointing to the north pole is more stable.
Nuclei behave similarly when placed in an external magnetic field. They can either be spin-aligned, meaning their spin is parallel to the magnetic field, or spin-opposed, meaning their spin goes against the magnetic field. Understanding spin is key to understanding NMR spectroscopy, which we'll explore more in this article.
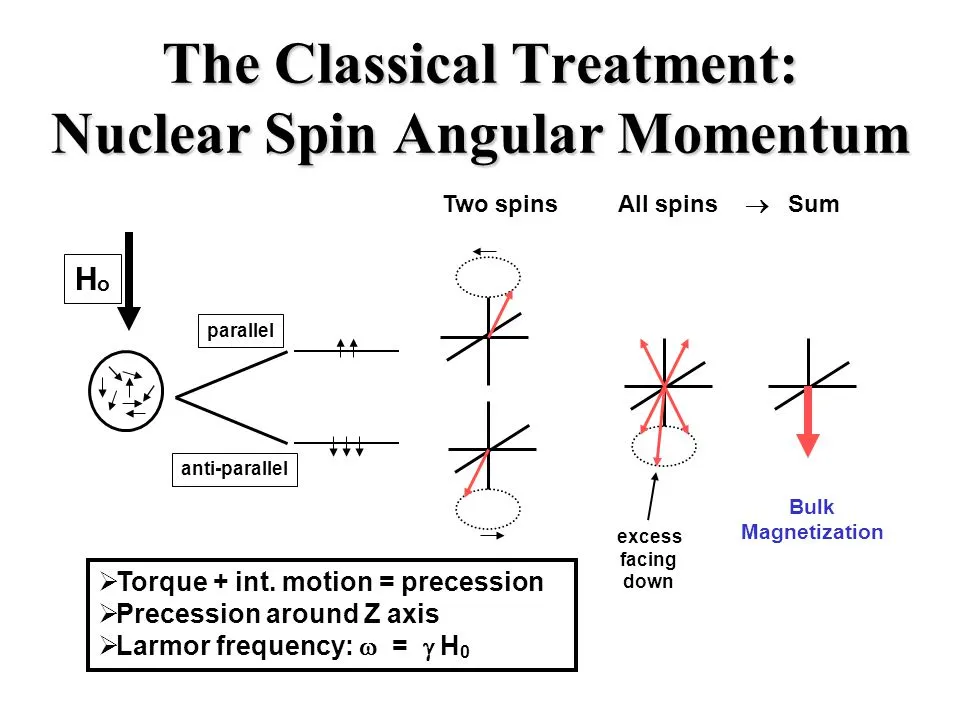
Resonance
The parallel (spin-aligned) state is much more stable than the antiparallel (spin-opposed) state. It therefore has lower energy. We call the energy difference between the two states ΔE.
We normally find most nuclei in the parallel state because it is more energetically stable. However, we can force nuclei to flip from parallel to antiparallel by supplying them with a certain amount of energy. This energy is equal to ΔE and varies according to the strength of the magnetic field surrounding the nuclei. We call this process of switching from the parallel to antiparallel state magnetic resonance. The energy needed to switch states is known as magnetic resonance frequency.
What determines magnetic resonance?
The magnetic field that a nucleus with spin experiences depends on the atoms or groups surrounding it. Only nuclei with spin have resonance, which happens when an atom's nuclei flip to the antiparallel state after being exposed to the right amount of energy. This resonance frequency is equal to ΔE, which depends on the magnetic field surrounding the nuclei.
But why do different atoms experience different magnetic fields? Electrons can actually shield or block out external magnetic fields, similar to how people can block out the wind. For example, if a carbon atom is bonded to an oxygen atom, the oxygen's strong electronegativity attracts the shared electrons towards itself, leaving the carbon atom to experience the magnetic field more strongly. This results in a higher resonance frequency. Understanding how different atoms affect resonance is key to using NMR spectroscopy to determine molecular structure.
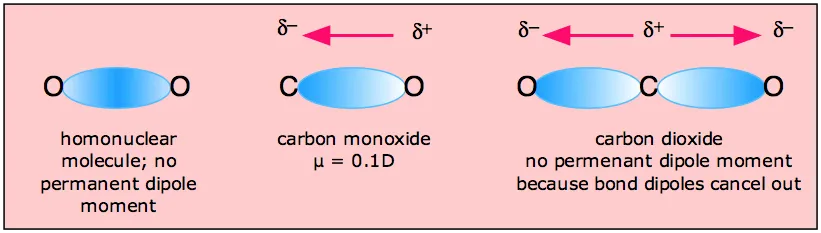
On the other hand, some groups of molecules are electron-releasing. These include methyl groups. If a carbon atom is bonded to three methyl groups, for example, it will have a lower resonance frequency than a carbon bonded to no methyl groups. This is because the electrons released by the methyl groups shield the nucleus from the magnetic field. They act like the other people, shielding the nucleus from the wind, and so the nucleus experiences the force of the field less strongly.
In summary: Each carbon atom needs a different amount of energy for it to flip to its antiparallel state, depending on the different atoms and groups attached to it. Carbon atoms attached to more electronegative groups have a higher resonance frequency than those attached to electron releasing groups.
What is the importance of resonance?
We can use computers to detect the resonance frequencies of different parts of a molecule and match them up to known structures. We supply energy to the sample of our molecule in the form of radio waves, and a detector detects the frequencies absorbed by the molecule. Because atoms attached to different groups have different resonance frequencies, we can then compare these frequencies to those of known structures in a database and work out what exactly the molecule looks like.
For example, the C=O bond found in aldehydes and ketones has a shift value of 190-220 ppm. We’ll explore what exactly that means later, but you should know that if we analyse a molecule using NMR spectroscopy and the results also include that value, there will be a C=O present.
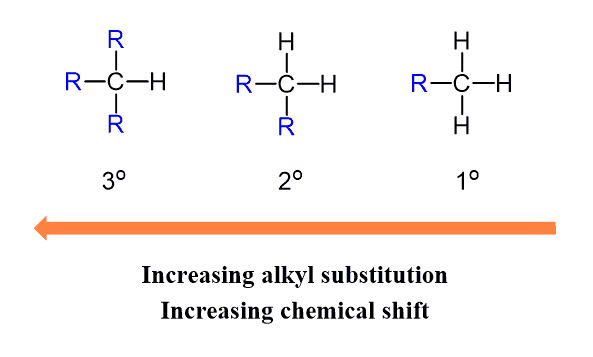
How do we carry out NMR?
To carry out NMR spectroscopy on an organic sample, we follow the following steps. Dissolve the sample in a suitable solvent. Add a small amount of tetramethyl silane. Apply radio waves so that some of the nuclei in the sample flip to their higher energy antiparallel state. Plot a graph showing the energy of the radio waves absorbed against chemical shift.
What is chemical shift?
Chemical shift is a quantity related to resonance frequency, which you know is the energy absorbed by the nuclei when they flip to their antiparallel state.
We give chemical shift the symbol δ and measure it in parts per million, or ppm. It is actually to do with the differences in resonance frequency between the nuclei in the sample and a reference molecule called tetramethyl silane. Also known as TMS, it gives a peak at the value 0 on the graph, and all other molecules take values greater than this. The more a nucleus is shielded from the magnetic field by electrons, the lower its resonance frequency and chemical shift value, so the nearer it’ll be to TMS in the spectrum. Confusingly, NMR graphs run from right to left. This means that TMS is always shown on the right-hand side of the spectrum.

What is tetramethylsilane?
Tetramethylsilane, or TMS, is a molecule that we use as a reference in NMR spectroscopy. As explained above, it has a chemical shift value of 0. All other nuclei have chemical shift values higher than this. TMS consists of a silicon atom bonded to four methyl groups, giving it the formula

Why do we use TMS?
TMS is useful as a reference molecule for the following reasons.
It has four carbon atoms in identical environments. We’ll explore the significance of this in the article Carbon-13 NMR, but you should know that this means it gives a strong, clear signal. It has a low boiling point and so is easy to remove from the sample after analysis is complete. It is inert and nontoxic. You don’t have to worry about it reacting with anything in your sample.
Types of NMR spectroscopy
We learnt that any atom with an odd mass number has nuclear spin and so can be used in NMR spectroscopy. However, this isn’t always very helpful when working out the structure of molecules. For example, what is the point of looking for peaks produced by atoms if your molecule only contains one chlorine atom? You might find out information about the groups bonded to that particular atom, but not the rest of the molecule! There are other atoms which are much more common in organic molecules - carbon and hydrogen.
Carbon-13 NMR
Carbon-13 is a less common isotope of carbon that has an atomic mass of 13 due to its seven neutrons. Despite only making up about 1% of all carbon atoms, carbon-13 is useful for NMR analysis in organic chemistry because it can provide information about different parts of a molecule. When using NMR spectroscopy, carbon-13 atoms produce peaks on the graph because they have an antiparallel state to flip to when exposed to the right amount of energy. Carbon-12 atoms, which make up the majority of carbon atoms, do not have nuclear spin and do not produce peaks on the graph. Carbon-13 NMR typically uses solvents and can produce peaks ranging from 0 to about 200. In the next section, we'll delve into carbon-13 NMR in more detail.
Proton NMR
There is another atom we can use in NMR that is even more common than carbon-13. is the main isotope of hydrogen, and its nucleus is actually just a proton. These nuclei give much smaller chemical shifts than carbon-13 nuclei, ranging typically from 0 to 10. Proton NMR spectroscopy, also known as hydrogen-1 spectroscopy, is a lot more useful than carbon-13 spectroscopy because the peaks produced are proportional to the number of nuclei in that environment. (For more detail, look at Hydrogen-1 NMR). Imagine that you have a methyl group, . There are three hydrogen atoms attached to the central carbon atom. Now imagine you have a group in the middle of a molecule. It has just one hydrogen atom attached. The three hydrogen atoms that are a part of the methyl group are all in the same environment and will produce a peak three times as high as the single hydrogen atom joined to the group.

Nuclear magnetic resonance spectroscopy is an analytical technique used to determine the structure of organic compounds. Nuclei with odd mass numbers have a property called spin, which makes them act like small bar magnets. When placed in an external magnetic field, they can be in a parallel or antiparallel state. The energy needed to flip a nucleus from its parallel state to its antiparallel state is called magnetic resonance frequency, which depends on how strongly the nucleus feels the external magnetic field. The groups or atoms surrounding the nucleus affect the strength of the external magnetic field, and better shielded nuclei have a lower resonance frequency. NMR graphs show chemical shifts against energy absorbed by the nuclei in a sample, using a TMS as a reference. Carbon-13 NMR and hydrogen-1 NMR, also known as proton NMR, are two common types of NMR spectroscopy.
Understanding NMR
How do you read NMR spectroscopy?
NMR spectroscopy produces graphs called spectra. Spectra show energy absorbed against a value called chemical shift. To read them, you look at the peaks produced on the graph and compare their chemical shift values to those in a data table to help you identify the molecule's structure.
What is the basic principle of NMR spectroscopy?
NMR spectroscopy uses a property called spin to identify molecular structure. Certain nuclei have spin and this makes them behave like bar magnets when placed in an external magnetic field. If you supply them with just the right amount of energy, they can flip from a spin-aligned state to a spin-opposed state. This energy varies depending on the other atoms or groups surrounding the nuclei. By plotting the energy absorbed by the nuclei on a graph, we can work out the possible structure of the molecule.
What does NMR tell you about a compound?
NMR tells you about the environment of certain nuclei in a compound. An atom's environment is all the other atoms and chemical groups surrounding it. This helps you work out the structure of the compound.
What are IR and NMR spectroscopy?
NMR spectroscopy is an analytical technique that uses a magnetic field to help work out the structure of a molecule. It shows you which other atoms or chemical groups surround certain nuclei in a compound. IR spectroscopy is another analytical technique that uses IR radiation to identify the chemical bonds in a molecule.
What is the purpose of NMR?
NMR spectroscopy is used to help work out the structure of a compound or molecule by identifying other atoms or chemical groups that surround certain nuclei.