Ion Colours
Transition metals are special because they form ions that have an incomplete d subshell. When they combine with other atoms or molecules, they create complex ions. These complex ions can be really cool because they come in all sorts of colours! You might recognize some of the colours if you've seen rust (iron oxide) or a copper roof turning blue-green. These colours can help us identify what kind of transition metal we're looking at.
But why do some complex ions have certain colours, and why do they sometimes change? We'll explore all of that in this article! We'll talk about where the colours come from, what affects them, and all the different colours that complex ions can have. We'll even learn about visible light spectroscopy and colorimetry, which are cool techniques for measuring coloured ions. Fun fact: Did you know that the Statue of Liberty in the USA is made of copper? It used to be a dull bronze colour, but over time it turned into the pale blue-green colour we see today! This is because of a process called oxidation, which happens when copper is exposed to the air for a long time. Some artists even use artificially aged copper because they like the blue-green colour it turns. The colour change of the Statue of Liberty is due to oxidation!
Factors affecting the colour of complex ions
To understand why transition metal complexes have different colours, we need to know about the electromagnetic spectrum. You might have heard that white light is made up of different colours. If you shine white light through a prism, you can see all the colours of the rainbow. This is because white light is actually made up of different wavelengths of light. The part of the spectrum that we can see is called visible light, but there are other types of light we can't see, like infrared and ultraviolet.
When light hits a transition metal complex, some of the light is absorbed by the complex. The energy from the absorbed light causes the electrons in the complex to move to a higher energy level. This is called an excited state. The amount of energy absorbed depends on the wavelength of light that hits the complex. The complex then reflects or transmits the remaining light, which is what we see as the complex's colour. The colour of a complex depends on several factors, including the metal ion, the ligands (the molecules or ions that surround the metal ion), and the oxidation state of the metal ion. Different combinations of metal ions and ligands can create different coloured complexes. And sometimes, a complex can change colour depending on its environment, such as changes in temperature or pH. Scientists use techniques like visible light spectroscopy and colorimetry to measure the amount of light absorbed by a complex and determine its concentration. So the next time you see a colourful transition metal complex, remember that its colour comes from the way it absorbs and reflects light!
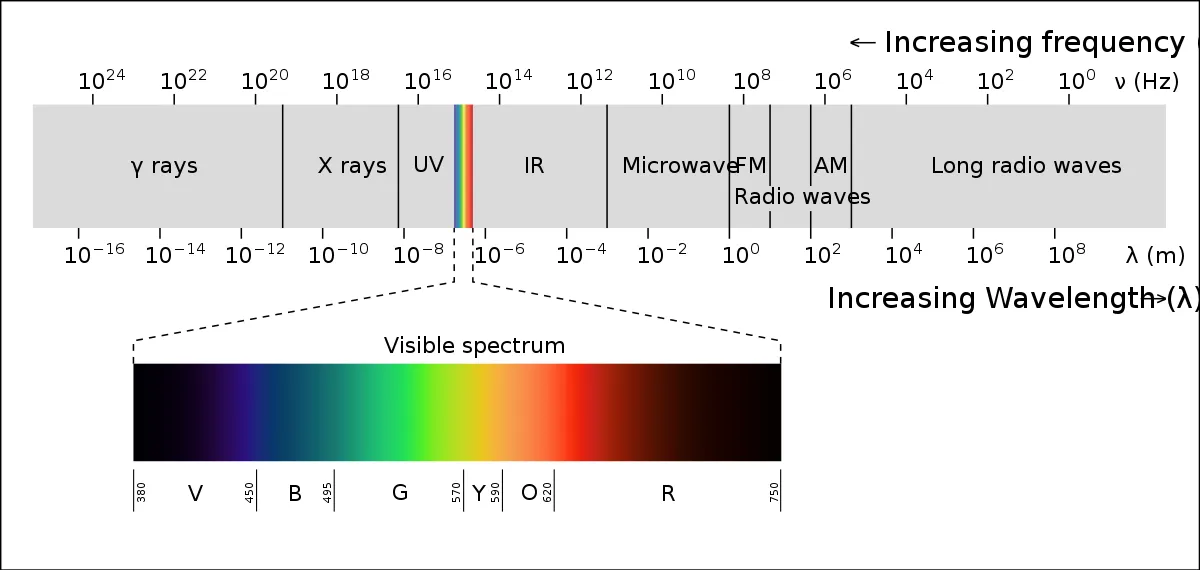
The visible spectrum has different colours that each have a specific wavelength. Red light has the longest wavelength at 700 nanometers, while violet light has the shortest at 400 nanometers. All the other colours are in between these two and have their own specific values. This means that there are countless colours in the visible spectrum!
When white light passes through a transition metal complex in water, it absorbs some of the wavelengths. The remaining wavelengths are either reflected or transmitted. These remaining wavelengths are what give the solution its colour. For example, when you look at a copper(II) sulphate solution, it appears cyan or pale blue. This is because the solution absorbs the light in the red region of the spectrum, so the light that comes out has all the other wavelengths except for red. When you mix all of these wavelengths together, you get the colour cyan.

You may be wondering what happened to the other colours not absorbed by the copper(II) sulphate solution. After all, an infinite number of colours exist in the visible spectrum. If the ion complex only absorbed red light, why do we see it as cyan blue, and not yellow or pink? We need to use a colour wheel like the one in the image below to find the answer.

In an octahedral complex, the five degenerate d-orbitals split into two sets of non-degenerate orbitals. The magnitude of the splitting, known as the ligand field splitting, determines which configuration is observed. The two sets of orbitals are known as the t2g and eg sets. The t2g set consists of three orbitals with lower energy and the eg set consists of two orbitals with higher energy. The t2g orbitals are oriented towards the ligands, while the eg orbitals are oriented towards the centre of the octahedron.
The colour of a transition metal complex is determined by the energy difference between the t2g and eg orbitals. If the energy difference is small, the complex will absorb light in the visible region and appear coloured. For example, if a complex absorbs red light, the light that passes through it will contain proportionately more of its complementary colour, i.e., blue. That is why copper(II) sulphate solution appears blue. The opposite of red on the colour wheel is blue, so when copper(II) sulphate absorbs red light, the light that gets transmitted will have more of its complementary colour, i.e., blue.
An octahedral complex is a complex that has six ligands covalently bonded to the central transition metal ion. In this type of complex, the degenerate 5 d-orbitals split into two levels with dx2-y2 and dz2 at a higher level and dyz, dxz, and dxy at a lower level. This happens because the lone pairs of electrons in the dx2-y2 and dz2 orbitals repel more than in the dyz, dxz, and dxy orbitals. This is because the ligands bind to the central metal ion along the x, z, and y axis, and the dx2-y2 and dz2 orbitals have lobes on these axes, making the electrons closer together and leading to more repulsion. The energy difference between the two levels is ΔE.
On the other hand, a tetrahedral complex has four ligands covalently bonded to the central transition metal ion. In this type of complex, the ligands line up with dyz, dxz, and dxy, meaning that there is more repulsion than at dx2-y2 and dz2. Therefore, dyz, dxz, and dxy will be at a higher energy level, while dx2-y2 and dz2 will be at a lower and more stable energy level compared to the former. The energy difference between the two levels is ΔE, as in the splitting in octahedral complexes.
Electron promotion
The energy difference between the two sets of orbitals determines which wavelengths of light the complex can absorb. When light shines on the complex, electrons in the lower energy level can absorb energy and jump to the higher energy level. The energy absorbed corresponds to a particular wavelength of light, which is complementary to the colour of the complex. The complex appears coloured because it absorbs certain wavelengths of light, leaving only its complementary colour to be transmitted to our eyes.
In the case of copper(II) sulphate, the complex absorbs light in the red region of the spectrum, giving it a complementary blue colour. This is because the energy difference between the two sets of orbitals corresponds to the energy of red light. When red light shines on the complex, electrons in the lower energy level absorb the energy and jump to the higher energy level, leaving only blue light to be transmitted to our eyes. This is why copper(II) sulphate solution appears blue. In contrast, zinc sulphate has a complete 3d subshell, so its ions do not have a partially filled 3d subshell. Consequently, it does not undergo ligand-induced splitting of its d orbitals, and it does not absorb any visible light. Therefore, zinc sulphate solution is colourless.

When the copper(II) sulphate solution absorbs light from the visible spectrum, an electron from the lower energy level gets promoted or excited by moving to the higher energy level. We say it moves from the ground state to an excited state. The energy absorbed by the excited electron depends on the difference in energy between the two levels.

Exactly! The small energy difference between the split 3d energy levels of the Cu2+ ion corresponds to the energy of red light, which is why copper(II) sulphate appears cyan blue, the complementary colour of red. In contrast, the completely filled 3d subshell of the zinc ion means that no splitting occurs, and no electrons get excited. As a result, zinc complexes are colourless.

How to find ∆E of an excited electron
That's correct! The equation you provided, E = hv, allows us to calculate the energy of a photon based on its frequency. When an electron moves from the ground state to an excited state, it absorbs a photon with a specific energy. The energy of the photon is proportional to its frequency, so by measuring the frequency of the absorbed light, we can determine the energy change of the electron.
The equation you mentioned, λ = c/v, allows us to calculate the wavelength of light based on its frequency. This equation is related to the energy of the photon, since the energy of a photon is also inversely proportional to its wavelength. As the wavelength of light decreases, the frequency and energy of the photon increase.
By using these equations, we can determine the specific wavelength of light that a complex absorbs, and therefore predict the colour of the complex. This is useful in many applications, such as in the food industry, where the colour of a product can indicate its quality or freshness.
Why do these ions change colour?
The oxidation state, ligands, and coordination number of an ion complex can all affect the level of splitting in the d orbitals, and therefore change the amount of energy absorbed by electrons and the resulting colour of the complex.
Firstly, the oxidation state of the central metal ion can have a significant impact on the colour of the complex. As the oxidation state of the metal ion increases, the level of splitting in the d orbitals generally increases as well. This means that the energy of the absorbed light also increases, resulting in a shift towards shorter wavelengths and bluer colours. For example, the Cu2+ ion has a partially filled d orbital that splits into two energy levels, leading to a characteristic blue colour. However, the Cu+ ion has a completely filled d orbital and does not display this splitting, leading to a colourless or pale yellow complex.
Secondly, the ligands that bind to the central metal ion can also affect the level of splitting in the d orbitals. Different ligands have different strengths and can cause varying degrees of splitting. Ligands that cause a small splitting, such as water or ammonia, tend to result in complexes with longer wavelengths and redder colours. In contrast, ligands that cause a large splitting, such as cyanide or carbon monoxide, tend to result in complexes with shorter wavelengths and bluer colours. Lastly, the coordination number of the complex can also influence the level of splitting in the d orbitals. As the coordination number increases, the level of splitting generally decreases. This is because the electrons in the d orbitals become more spread out and experience less repulsion from each other. As a result, the energy of the absorbed light also decreases, leading to a shift towards longer wavelengths and redder colours. Overall, understanding how these factors affect the colour of ion complexes is important in many fields, from chemistry to materials science and beyond.
Oxidation state
That's a great summary! The oxidation state of an ion is indeed related to the number of electrons that the ion has gained or lost compared to its neutral state. For example, the Fe2+ ion has lost two electrons and has an oxidation state of +2, while the Fe3+ ion has lost three electrons and has an oxidation state of +3.
As you mentioned, transition metals have unique properties that allow them to have varying oxidation states. This is due to the presence of partially filled d orbitals, which can accept or donate electrons to form different oxidation states. As the oxidation state of the metal ion increases, the splitting in the d orbitals generally increases as well. This is because the electrons in the d orbitals experience more repulsion from each other as the positive charge on the metal ion increases. The increased splitting results in a larger energy gap between the split orbitals, which in turn affects the colour of the ion complex. For example, the Mn2+ ion has a partially filled d orbital that splits into two energy levels, leading to a characteristic pink colour. However, the MnO4- ion has a completely filled d orbital and does not display this splitting, leading to a colourless or pale yellow complex. This is because the oxidation state of the Mn ion has changed from +2 to +7, leading to a large increase in splitting and a shift towards shorter wavelengths and bluer colours. Understanding how the oxidation state of a metal ion affects the colour of the ion complex is important in many fields, from materials science and engineering to biochemistry and medicine.
Ligands
Ligands are atoms or molecules that bind to a central metal ion in a complex ion. When ligands attach to the central metal ion, the 3d orbitals of the metal ion split into two sets of non-degenerate orbitals, as you mentioned. This is because the ligands exert an electrical field on the metal ion, which causes the energy levels of the d orbitals to split into two sets with different energies.
The size of the energy gap between the split 3d orbitals is determined by the strength of the electrical field surrounding the ligands. The stronger the electrical field, the larger the energy gap between the split orbitals. This energy gap determines the size of the light wavelength that an ion complex can absorb, and therefore affects the colour of the complex. Different ligands have different strengths and can cause varying degrees of splitting. For example, ammonia ligands have a stronger electrical field than water ligands, which causes more splitting in the 3d orbitals of a copper(II) ion complex. This causes the complex to absorb light at longer wavelengths, resulting in a deeper navy blue colour. In contrast, water ligands cause less splitting in the 3d orbitals, resulting in light absorption at shorter wavelengths and a lighter blue colour.
Overall, the identity of the ligands attached to a complex ion can have a significant impact on the colour of the complex. Understanding how different ligands affect the splitting of the 3d orbitals can help predict the colour of a complex ion and optimize its use in various applications.

Coordination number
That's a great explanation! The coordination number is indeed the number of ligands attached to the central metal ion in a complex ion. Changing the coordination number can affect the splitting of the 3d orbitals and therefore change the colour of the complex ion.
In the example you provided, the reaction between copper(II) sulfate solution and concentrated hydrochloric acid involves a change in the coordination number from 6 to 4. This means that the original 6 water ligands have been replaced by 4 chloride ion ligands. This change in ligands affects the splitting of the 3d orbitals and causes a change in the colour of the complex ion from blue to green to yellow.
It's important to note that changing the coordination number usually involves changing the ligands attached to the central metal ion, so it's difficult to isolate the effect of coordination number on the colour of the complex ion. However, understanding how changes in coordination number affect the splitting of the 3d orbitals can help predict and explain changes in colour observed in complex ions.
Visible light spectroscopy
Spectroscopy is a branch of chemistry that studies the absorption and emission of all kinds of radiation and light from the electromagnetic spectrum, while colorimetry is a type of spectroscopy that involves light from the visible spectrum only.
In visible light spectroscopy, different frequencies of light are passed through a sample of complex ions in solution, and the amount of light absorbed by the sample is measured using a detector and recorder. The filter used must match the part of the spectrum that the coloured complex absorbs the most, so that maximum absorption can take place.
A colorimeter is a simple device that measures the amount of visible light absorbed by a solution. The amount of light absorbed depends on the concentration of the coloured ions in solution, so a colorimeter can be used to determine the concentration of these ions. By using spectroscopy and colorimetry, chemists can identify substances based on their absorption and emission spectra, as different substances absorb and emit different wavelengths of light. These techniques are widely used in fields such as analytical chemistry, biochemistry, and materials science.

Once you have plotted the values on a calibration graph and drawn a line of best fit, you can use this graph to determine the concentration of an unknown sample solution of [Cu(H2O)6]2+ ions by measuring its absorbance and comparing it to the calibration curve.
For example, let's say you measure the absorbance of your unknown sample solution of [Cu(H2O)6]2+ ions and get a value of 0.120. You can use the calibration curve to determine the concentration of the sample solution as follows:
- Find the point on the calibration curve that corresponds to an absorbance of 0.120.
- Draw a horizontal line from this point to the y-axis (concentration axis) to find the concentration of the sample solution.
- In this case, the concentration of the sample solution is approximately 0.36 mol dm-3.
So, by using a calibration graph and measuring the absorbance of an unknown sample solution of [Cu(H2O)6]2+ ions, you can determine its concentration and accurately identify the substance.
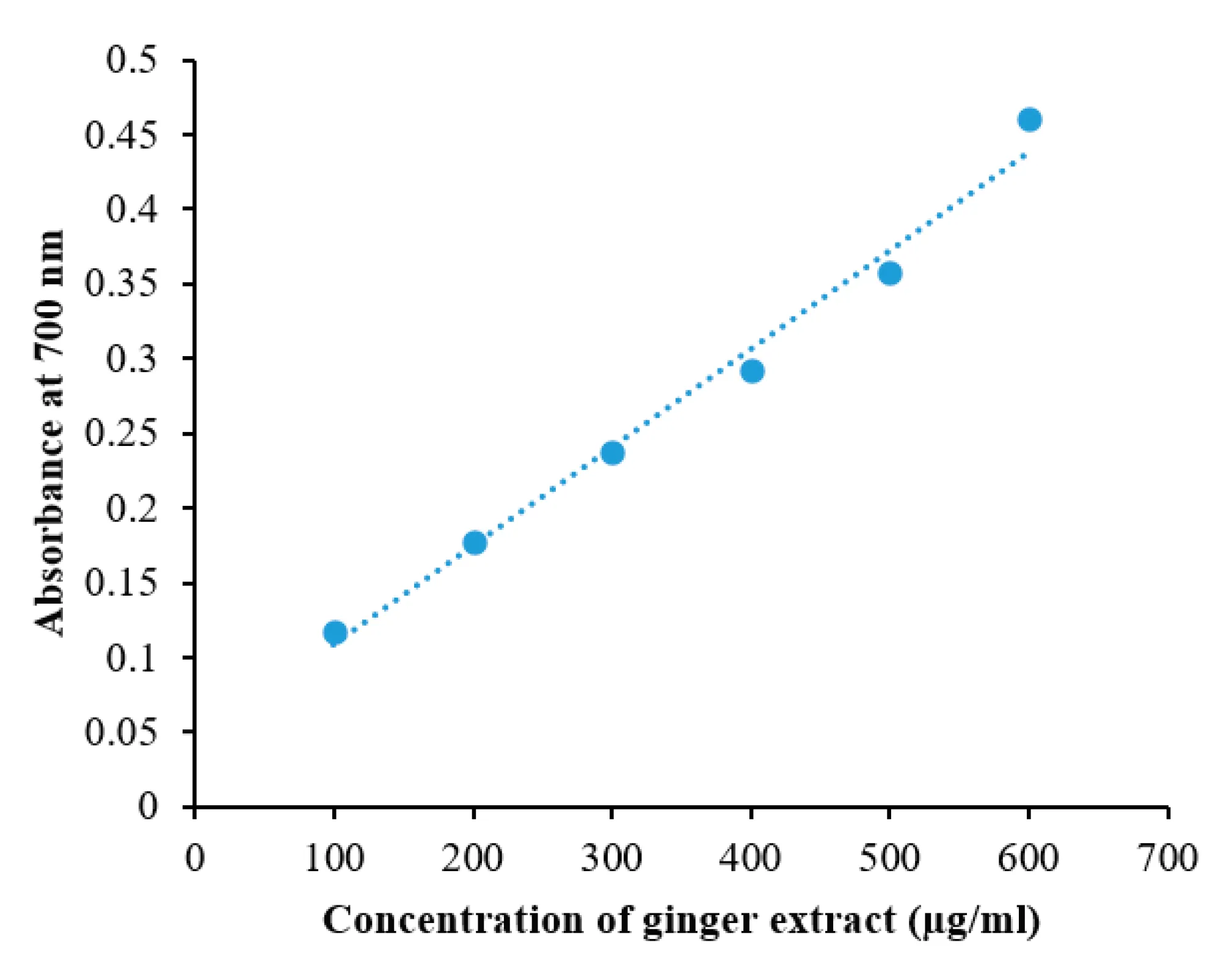
Sure, let's take a look at the colors of some common ion complexes.
The ferric ion, Fe3+, forms a complex ion with water, [Fe(H2O)6]3+. This complex ion appears yellow-brown in solution.
The manganese ion, Mn2+, forms a complex ion with water, [Mn(H2O)6]2+. This complex ion appears pale pink in solution.
The chromium ion, Cr3+, forms a complex ion with water, [Cr(H2O)6]3+. This complex ion appears green in solution.
These colors are due to the specific wavelengths of light that are absorbed by each complex ion. By using spectroscopy and colorimetry, we can identify these and other complex ions based on their unique absorption and emission spectra.
The colour of the ferric ion
The chemistry of iron can be quite complex, but it is also fascinating! The ability of iron to switch between its oxidation states is essential for many biological processes, including oxygen transport and storage in our bodies. It is amazing how our bodies have evolved to control the concentration and distribution of iron ions in order to maintain proper health and function.
The colour of the permanganate ion
Permanganate is the name we give compounds that have manganese in the +7 oxidation state. The permanganate ion (MnO4-) is a vibrant purple colour. Be careful not to confuse permanganate with manganate, which is the word we use for manganese in the +6 oxidation state. Manganate appears green in solution.
The colour of chromium ions
The colors of complex ions can be truly remarkable and have practical applications in a variety of fields, from art and fashion to medicine and electronics. However, as you mentioned, it is important to be aware of the potential toxicity of some metal ions and their compounds, such as chromium(VI). This is why it is crucial to use safe and responsible practices when handling and disposing of these substances.

Understanding the principles of ion colors and their measurement is essential for anyone working in fields related to chemistry, physics, and materials science. By being able to accurately determine the concentration of colored ions in solution, researchers can gain important insights into the behavior of these substances and develop new applications for them. Furthermore, by understanding the factors that affect ion colors, we can create new materials with specific optical properties and use them in a wide range of applications, from solar cells and LEDs to sensors and imaging devices.
Ion Colours
What determines the colour of an ion?
The amount of light energy absorbed (∆E) by electrons in the split d orbital gives ion complexes their unique colours. Different colours of light have different wavelengths and a corresponding light frequency. The energy gap between the split d orbitals determines the frequency of light an electron can absorb.
Why are some ions coloured?
The colour of transition metal ions comes from their incomplete 3d subshell. Ligands that attach themselves to the central ion cause the 3d orbitals to split into two sets with different energy levels. The energy gap between the split orbitals allows excited electrons to absorb different wavelengths of light from the visible spectrum. The rest of the light passes through and gives the transition metal its colour.