Benzene Structure
Benzene is a type of hydrocarbon that has a unique structure. It has six carbon atoms and only six hydrogen atoms, which makes it different from most other hydrocarbons. Despite its unusual structure, benzene is very common and important in our daily lives.
You can find benzene in many of the foods we eat, like vanilla-flavored treats. Benzene is also a key ingredient in ibuprofen, a common pain reliever. And if you've ever smelled fresh paint, you've experienced another type of benzene called toluene.
Benzene is known as an aromatic compound, which means it has a special ring of electrons that are spread out, or "delocalized." This is something you might have learned about in your Aromatic Chemistry class. We'll dive deeper into the structure of benzene in the next section. So stay tuned! And remember, benzene is everywhere, even in the air we breathe!

How is benzene structured?
Benzene has a unique structure. There are some important structural aspects you need to know about, such as its formula, bond lengths, and electron arrangement.
Structural and displayed formulae
As we mentioned above, benzene has the molecular formula. It forms a hexagonal molecule, which we often represent as a hexagon with a circle inside.
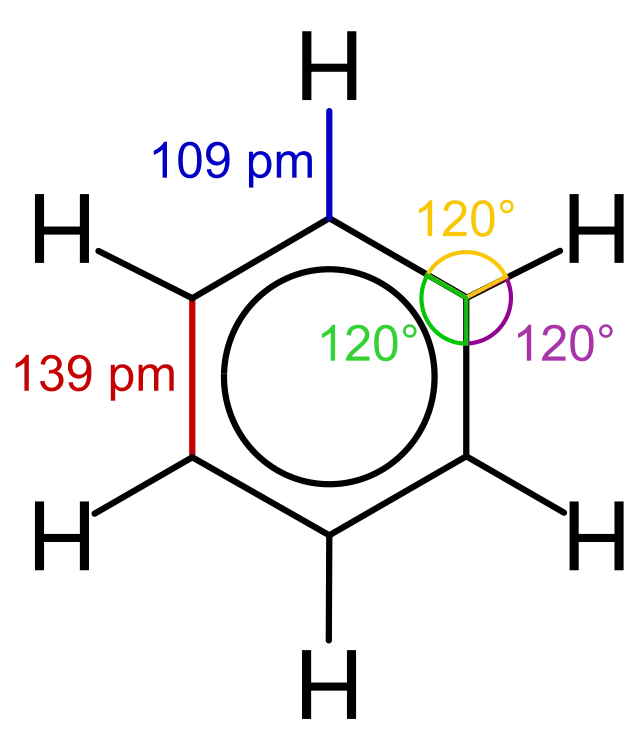
Bond length
We explored some potential structures for benzene in Aromatic Chemistry, each containing three C=C double bonds. But in actual fact, we know that benzene doesn’t contain any double bonds at all. Instead, all of its carbon-carbon bonds are identical intermediates - halfway between a single and a double bond in length. We’ll explore why in just a second.
Bond angle
Each carbon atom in benzene is bonded to two other carbon atoms and just one hydrogen atom, and the bond angle between each bond is 120°. This makes benzene a trigonal planar molecule.
We know that carbon has four valence electrons, which means it can form bonds with up to four other atoms. In the case of benzene, only three of the carbon's valence electrons are used to form bonds with other atoms. So, what happens to the fourth electron?
This is where the concept of electron orbitals and the delocalised pi system comes into play. In benzene, the fourth electron is in an orbital that is not involved in bonding. Instead, it is part of a ring of electrons that are spread out, or "delocalised," over all six carbon atoms in the benzene ring. This is known as the delocalised pi system. The delocalised pi system is what gives benzene its unique properties, including its stability and resistance to reactions. It also contributes to the characteristic smell of benzene and its derivatives. So, while benzene may have an unusual structure, it is this very structure that makes it so important and interesting in the world of chemistry.
The delocalised pi system
Carbon’s fourth outer-shell electron is found in a pi orbital, whereas the bonded three are found in sigma orbitals. Sigma orbitals stretch between atoms whilst pi orbitals extend above and below the atom. In benzene, all the pi orbitals of the carbon atoms overlap, producing a connected region that stretches above and below the molecule.
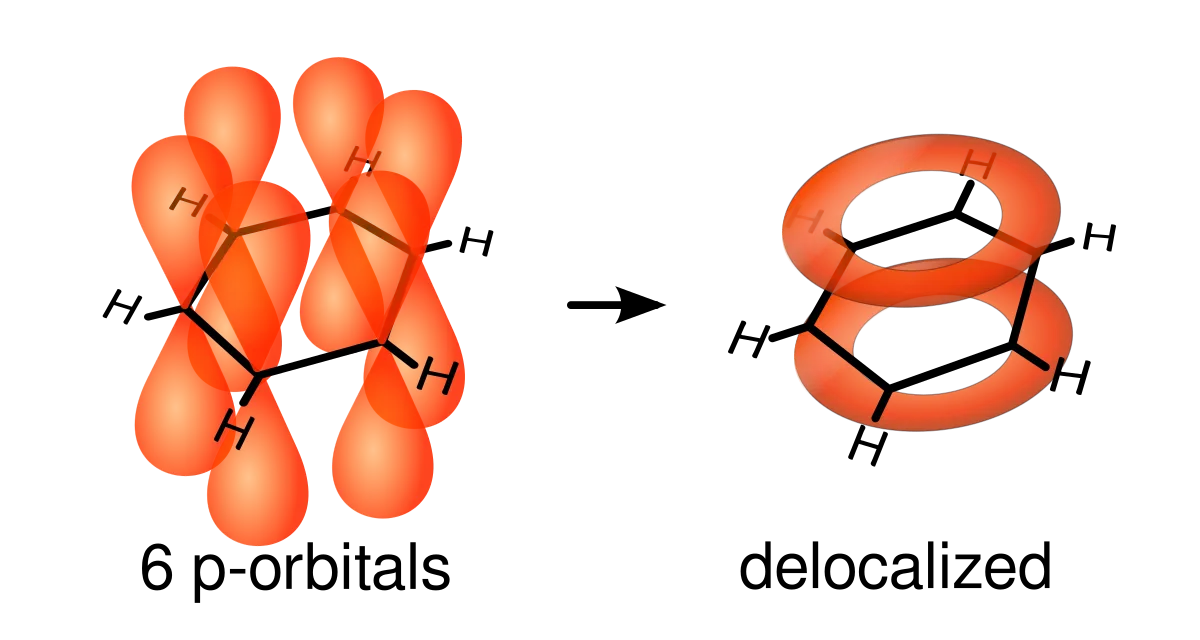
When we look at the structure of benzene, we can see that its delocalised pi system is what makes it unique. The electrons are not confined to specific bonds, but can move freely throughout the overlapping region, giving benzene its stability and resistance to reactions.
Because of this delocalisation, benzene does not need to form any double bonds. Instead, all of its C-C bonds are identical intermediates, halfway between a single and double bond in length. This results in a planar hexagonal shape with an angle of 120° between bonds.
In 1865, Friedrich August Kekulé proposed a different structure for benzene, based on his dream of a snake biting its own tail. He suggested that benzene contained alternating C-C single and C=C double bonds, as shown in cyclohexa-1,3,5-triene. However, there were pieces of evidence that did not support this structure.
Despite Kekulé's proposal, the delocalised pi system is widely accepted as the correct structure for benzene. It is this structure that gives benzene its unique properties and makes it so important in the world of chemistry.
Electrophilic addition reactions
That's correct. Kekulé's structure for benzene contains three C=C double bonds, so if it were true, we would expect it to undergo electrophilic addition reactions with substances like bromine water. However, as you mentioned, benzene does not react with bromine water in this way. Instead, the solution remains red-brown, indicating that no electrophilic addition reaction has taken place. This observation was one of the key pieces of evidence that led to the rejection of Kekulé's structure for benzene. Instead, chemists came to realize that benzene has a delocalized pi system, which means that its electrons are not confined to specific bonds but rather spread out over the entire molecule. This delocalization makes benzene much less reactive than expected based on its structure, and explains why it does not undergo the expected electrophilic addition reactions with substances like bromine water.
Enthalpy of hydrogenation
Reactions that add hydrogen to a molecule are known as hydrogenation reactions. Let’s look at cyclohexene, shown below. It has an enthalpy of hydrogenation of , meaning that of energy are released when two hydrogen atoms add on to its single double bond. This produces cyclohexane.
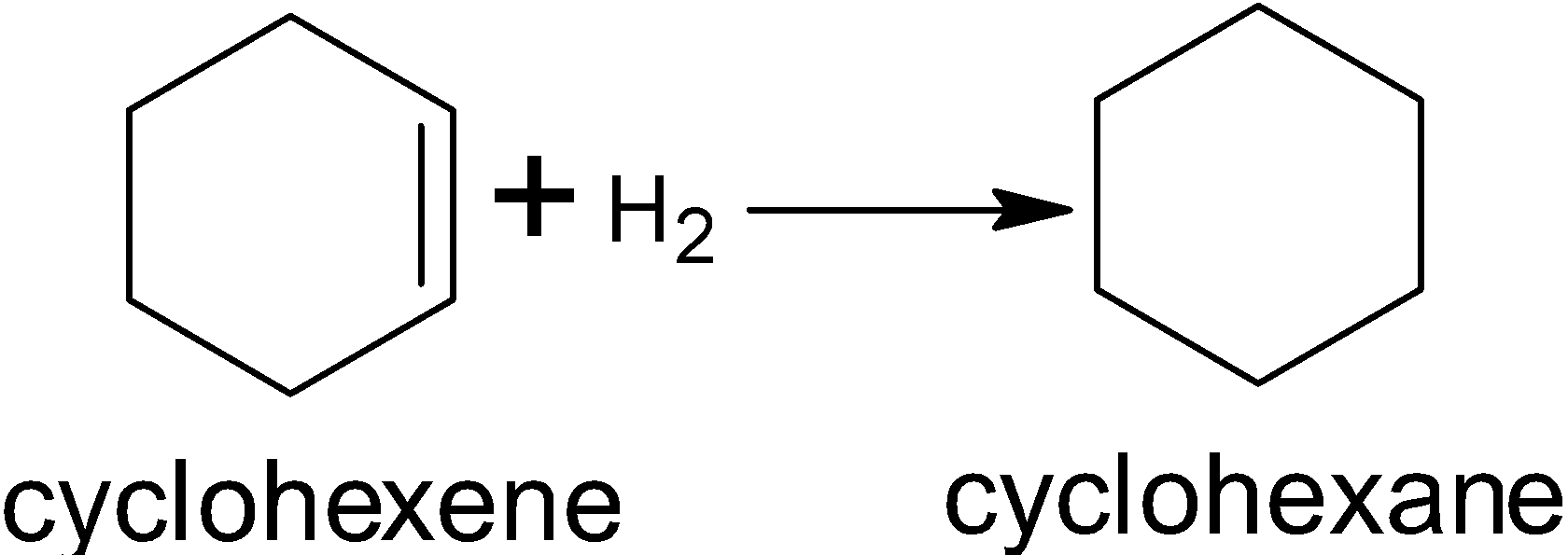
The evidence for the enhanced thermodynamic stability of benzene was obtained from measurements of the heat released when double bonds in a six-carbon ring are hydrogenated to give cyclohexane as a common product. The heat of hydrogenation of an alkene is the standard enthalpy of catalytic hydrogenation of an alkene, and is always negative. The standard enthalpy of the reaction for cyclohexene is -30.3 kcalmol-1, which is three times less than the -38 kcalmol-1 for benzene. This difference in enthalpy is known as benzene's resonance energy, and is due to its ring of delocalized electrons, which stabilizes the molecule by spreading the electrons' negative charges over a larger area.
Bond lengths
X-ray diffraction is a type of technique using X-rays to work out the structure of molecules. Scientists used it in 1981 to get an image of benzene. In Aromatic Chemistry, we learnt that C-C single bonds are longer than C=C double bonds. This would give Kekulé’s cyclohexa-1,3,5-ene a distorted shape.
However, the image showed that benzene was in fact a regular hexagon. This meant that all of its bonds were equal length. Furthermore, scientists measured the length of these bonds and found them to be halfway between a single and a double bond in length - suggesting that they were neither one nor the other, but something different instead.
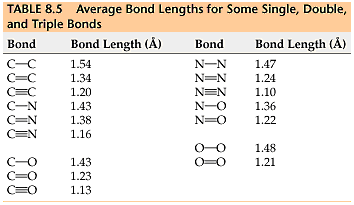
Isomeric products
Let’s look at one final piece of evidence against Kekulé’s predicted structure of benzene. Take two of benzene’s adjacent carbon atoms. Imagine swapping the attached hydrogen atoms for bromine, for example. If benzene really was cyclohexa-1,3,5-triene, we’d expect it to form two different isomers: one with a double bond between the two affected carbons, and one with a single bond between them. We can see this below.

However, scientists only ever observed one isomer. This meant that benzene couldn’t have Kekulé’s predicted structure. It had to have identical bonds.
Poor Kekulé - he really thought he had cracked the mystery of benzene, but all the evidence was against him! He proposed one final idea - benzene consisted of two structures in equilibrium, rapidly shifting between both. This would result in a hybrid molecule that was neither one nor the other. He called this the resonance model. However, there was no evidence to support this. We instead now believe in the delocalised model described earlier. The delocalisation accounts for benzene’s resonance energy and the identical intermediate C-C bonds explain why benzene is a regular shape.

The properties of benzene
Because of its unique structure and ring of delocalisation, benzene has some unique properties. Let’s explore them below.
Combustion
You should know that cyclic alkanes have a general formula . A cyclic hydrocarbon with six carbon atoms would therefore have twelve hydrogen atoms. However, benzene has six carbon atoms but only six hydrogen atoms. This higher ratio of carbon to hydrogen means benzene burns with a characteristically sooty flame.
Melting and boiling point
You are correct that the intermolecular forces between molecules of benzene are weak van der Waals forces. However, the planar structure of benzene allows for a phenomenon known as pi-stacking, which occurs when the pi electrons in neighboring molecules interact with each other. This interaction leads to stronger intermolecular forces than would be expected based solely on the London forces, and can contribute to the neat packing of solid benzene.
Cyclohexane, on the other hand, is a nonpolar molecule with a tetrahedral geometry, as you mentioned. This geometry results in a less efficient packing arrangement in the solid state than benzene, and therefore weaker intermolecular forces. This is why the melting point of benzene is higher than that of cyclohexane.
In the liquid state, however, the neat packing arrangement of benzene is disrupted, and the strength of the intermolecular forces between the two molecules becomes comparable. This is why the boiling points of benzene and cyclohexane are similar.

Solubility
Like other nonpolar hydrocarbons, benzene is insoluble in water but soluble in other organic solvents.
Reactivity of benzene
You are correct that benzene prefers to undergo substitution reactions rather than addition reactions due to the stability of its delocalized electron system. In substitution reactions, one atom or group is replaced by another without disrupting the delocalized system. The most common substitution reaction of benzene is electrophilic aromatic substitution.
In electrophilic aromatic substitution, an electrophile (an electron-deficient species) is attracted to the electron-rich ring of benzene and forms a covalent bond with one of the carbon atoms. This results in the removal of one of the hydrogen atoms from the ring, which is replaced by the electrophile.
The high electron density of the benzene ring makes it a very attractive target for electrophiles, which are often positively charged or electron-deficient. Common electrophiles used in electrophilic aromatic substitution include halogens (such as chlorine or bromine), nitro groups, and carbocations.
Overall, the stability of the delocalized electron system in benzene makes it less reactive than other unsaturated hydrocarbons, but also makes it a prime target for electrophilic substitution reactions.
What are electrophiles?
Electrophiles are electron pair acceptors. As the term -phile comes from the Latin philos, meaning love, we can say that they really just love electrons! Electrophiles have a positive or partial positive charge and a vacant orbital. Some common examples are and .
Electrophilic substitution reactions
You have provided a comprehensive summary of the key takeaways regarding the structure and reactivity of benzene. It is important to note that the stability and reactivity of benzene are due to its unique structure, which allows for the delocalization of pi electrons and makes it a prime target for electrophilic aromatic substitution reactions.
Nitration and Friedel-Crafts acylation are two common examples of electrophilic substitution reactions that benzene undergoes. These reactions involve the replacement of a hydrogen atom on the benzene ring with a nitro group or acyl group, respectively, resulting in the formation of a substituted benzene derivative. These derivatives are often used in the production of dyes, pharmaceuticals, plastics, and detergents. Overall, the properties and reactivity of benzene make it a crucial molecule in the field of organic chemistry, with numerous applications in various industries.
Benzene Structure
How many resonance structures does benzene have?
Benzene has two different resonance structures.
How do you draw the resonance structures of benzene?
To draw the resonance structures of benzene, draw a hexagon shape connected by alternating single and double bonds.
What is the structure of benzene?
Benzene is a planar hexagonal molecule made from six carbon atoms and six hydrogen atoms. Each C-C bond is an intermediate, halfway between a single and a double bond in length. One electron from each carbon atom is delocalised, and is found delocalised in overlapping pi orbitals above and below the planar carbon ring.
Why is benzene so reactive?
Benzene is actually relatively unreactive. Its delocalised pi electrons distribute the charge evenly around the molecule which stabilises it, making it less likely to react.
What is structure in organic chemistry?
Structure in organic chemistry is the arrangement of atoms, electrons and bonds in organic molecules, which are molecules based on carbon and hydrogen.