Properties of Transition Metals
Transition metals have some unique and interesting properties. In this article, we will explore some of these properties. Don't worry if it sounds complicated, we'll break it down for you. First, let's define what a transition metal is according to the International Union of Pure and Applied Chemistry (IUPAC). Simply put, a transition metal is an element whose atoms have partially filled d orbitals or can form stable cations with incompletely filled d orbitals.
Common properties of transition metals
Transition metals have a variety of properties that make them unique. These include their atomic radius, which is the distance from the center of the nucleus to the outermost electron. They also have high melting and boiling points, which means they can withstand high temperatures. In addition, they have a strong metallic character, meaning they can conduct heat and electricity well.
Transition metals also have a variable oxidation state, which means they can have different charges and form different compounds. They are also known for their ability to form complex ions and coloured compounds. Lastly, they have catalytic activity, which means they can speed up chemical reactions without being consumed in the process. It may seem like a lot to take in, but if you take your time and understand the logic behind each property, it will become easier to remember.
Atomic and ionic radius
The atomic radii of transition metals are smaller than those of s-block elements and larger than those of p-block elements. The atomic radius of 3d-series elements decreases initially from scandium to chromium, remains almost constant from chromium to copper, then increases towards the end of the series. This trend can be explained by the shielding effect, which refers to the repulsion of one electron by other electrons in multi-electron atoms. As the atomic number increases, the atomic radius decreases because the increase in nuclear charge outweighs the small shielding effect of d-shell electrons. As the number of d-electrons increases, the screening effect counterbalances the increased nuclear charge, resulting in an almost constant atomic radius. At the end of each period, the electron-electron repulsion between the added electrons in the same orbital is greater than the attractive forces due to the increased nuclear charge, resulting in the expansion of the electron cloud and an increase in atomic radius. Additionally, the ionic radius decreases with an increase in oxidation number and generally decreases with an increase in nuclear charge for the same oxidation state.
Melting point
The high melting points of transition metals can be attributed to the greater number of electrons from the d-subshell being involved in metallic bonding in addition to the s-electrons. This results in stronger metallic bonding, which requires more energy to break, thus leading to high melting points.
In any row of transition metals, the melting points rise to a maximum at the metal with electron configuration d5 (except for the anomalous values of Mn and Tc), then fall regularly as the atomic number increases. This can be explained by the fact that elements with d5 electron configuration have a half-filled d-subshell, which results in greater stability due to exchange energy. As a result, they have stronger metallic bonding and hence higher melting points.
However, the anomalous values of Mn and Tc can be attributed to their electron configurations. Mn has a half-filled d5 and a completely filled d10 subshell, which results in stronger metallic bonding and hence a higher melting point than expected. Tc has a half-filled d5 and a partially filled d6 subshell, which results in weaker metallic bonding and hence a lower melting point than expected.
In summary, the high melting points of transition metals can be attributed to their strong metallic bonding, which involves both s- and d-electrons. The trend in melting points across a row of transition metals rises to a maximum at the metal with d5 electron configuration, except for Mn and Tc, which have anomalous values due to their unique electron configurations.
Enthalpy of atomisation
The enthalpy of atomisation (∆Hat) is the enthalpy change when 1 mole of gaseous atoms is formed from its element under standard conditions
In general, the greater the number of valence electrons, the stronger the resultant bonding. Metals with a very high enthalpy of atomisation (i.e., very high boiling point) tend to be relatively unreactive for this reason. The metals of the second and third series have greater enthalpies of atomisation than the corresponding elements of the first series. This is an important factor in accounting for the occurrence of much more frequent metal-metal bonding in compounds of heavy transition metals.
Metallic character
All the transition elements are metals. They exhibit all the characteristics of metals. They all have high density, hardness, high melting points and boiling points, high tensile strength, ductility, malleability, high thermal and electrical conductivity.
Ionisation enthalpy
The first ionisation enthalpy of d-block elements lies in between that of s- and p-block elements. The ionisation enthalpy gradually increases with an increase in atomic number along a given transition series, though some irregularities are observed.
The first ionisation enthalpies of 3d-series elements are given below:
ElementScTivCrMnFeCoNiCuZnFirst ionisation enthalpy631656650653717762758736745906
The increase in ionisation enthalpy is due to an increase in nuclear charge with an increase in atomic number. This reduces the size of the atom making the removal of outer electrons more difficult. In a given series, the difference in the ionisation enthalpy between any two successive d-block elements is much less than the difference in the case of successive s- and p-block elements. The addition of d-electrons in the d-subshell with an increase in atomic number provides a screening effect. This shields the outer s-electrons from the nuclear pull. This means that the effect of increased nuclear charge and addition of d-electrons tend to oppose each other. Due to this, the ionisation enthalpy shows little variation on moving along a period of d-block elements. The first ionisation enthalpies of Zn, Cd, and Hg are however very high, because of the completely filled d10 s2 electron configurations.
Variable oxidation states
Transition metals exhibit variable oxidation states. The variable oxidation states of transition metals are due to similar energy levels of the s- and d-orbitals. Oxidation states of the first transition elements are given below. Oxidation states within brackets are unstable, while the most common oxidation states are in bold type.
Magnetic properties of transition metals
The magnetic properties of a compound are a measure of the number of unpaired electrons it contains. When a magnetic field is applied, we notice that there are two types of substances.
Paramagnetic substances: the substances which are attracted by a magnetic field are called paramagnetic substances. This character arises due to the presence of unpaired electrons. Diamagnetic substances: The substances which are repelled by a magnetic field are called diamagnetic substances. This character arises due to the fact that all the electrons are paired.
Most of the transition ions or their compounds have unpaired electrons in the d-subshell. Therefore, they are paramagnetic in character. The magnetic character is expressed in terms of the magnetic moment. The larger the number of unpaired electrons in a substance, the greater the paramagnetic character, and the larger the magnetic moment.
The magnetic moment is expressed in Bohr magneton, abbreviated as B.M. It is calculated by using the spin only formula:
µ = √n(n+2)
Where n is the number of unpaired electrons andµ is the magnetic moment. The magnitude of this magnetic moment is very small and is measured in the unit called Bohr magneton, μB. It is equal to 9.27 × 10–24 J T-1.
There's an interesting property which diamagnetic materials possess - if they are placed in a strong enough magnetic field, they levitate. In 1997, a group of scientists in England and the Netherlands, made a frog levitate in a strong magnetic field (16 teslas). This was possible since living organisms are made up of organic matter which is weakly diamagnetic. Since diamagnetic objects are repelled by a magnetic field, they levitate in order to get away from it. If you're now curious to see this floating amphibian, look up levitating frog on YouTube. it is truly fascinating!
We talked a bit about orbitals in this article. Most of the transition metals have a d orbital as the outermost orbital. Orbitals are defined by mathematical functions that give a probability of finding an electron in a certain region around the nucleus. There are 5 d orbitals - dxy, dyz, dxz, dz2, dx2-y2.
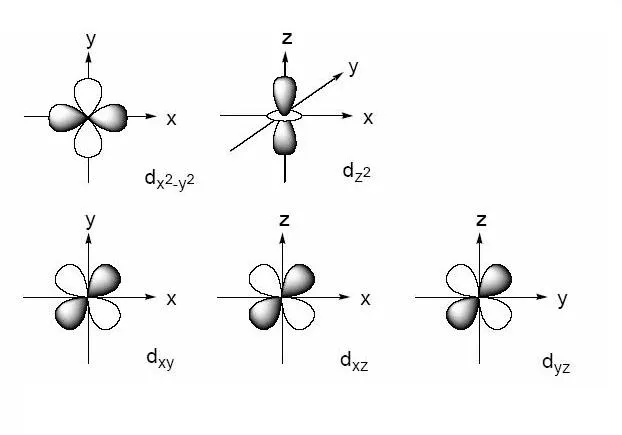
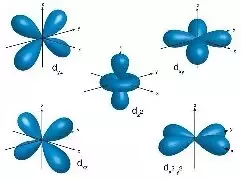
Formation of complex compounds
The transition metals and metal ions form a large number of complex compounds. In these compounds, a central transition metal ion is bonded to the number of ions or neutral molecules by co-ordinate bonds. Such ions or neutral molecules are called ligands. This is due to the following reasons: Small atomic size and ionic size.High nuclear charge. Availability of vacant d-orbitals to accept one pair of electrons donated by ligands.
Catalytic activity
The use of transition metals as catalysts is widespread due to their unique properties, such as their ability to exhibit variable oxidation states, form unstable intermediate compounds and provide an alternative path for the reactant with lower activation energy, provide a large surface area for the adsorption of the reactant, and form dative bonds with ligands. These properties make them ideal for catalyzing a wide range of reactions, from small-scale organic synthesis to large-scale industrial processes.
The importance of chemical catalysis is evident from the number of Nobel prizes awarded for achievements related to it. The work of Paul Sabatier and Victor Grignard, Fritz Haber, Karl Ziegler and Giulio Natta, and many others have revolutionized the field of catalysis and have paved the way for numerous industrial processes that are essential to modern life. One of the most important reactions in large-scale organic synthesis is the Suzuki reaction, which is a palladium-catalyzed substitution that couples an organohalide with a boronic acid or boronate ester to produce polyalkenes, substituted biphenyls, and styrenes. This reaction is highly advantageous due to its ability to use water as a solvent, minimizing the use of toxic solvents and producing non-toxic side-products that can be easily removed from the reaction mixture. In summary, the catalytic activity of transition metals is due to their unique properties, which make them ideal for catalyzing a wide range of reactions. The numerous Nobel prizes awarded for achievements related to chemical catalysis highlight the importance of this field in advancing scientific research and industrial processes.
Formation of coloured ions
The properties of transition metals are unique and make them important in many fields, such as chemistry and engineering. One of the most notable properties of transition metals is that they exhibit variable oxidation states, which allows them to form a wide range of compounds with different properties and reactivities.
Another important property of transition metals is their tendency to be paramagnetic, which means that they are attracted to a magnetic field. This property is due to the presence of unpaired electrons in their d-subshells. One of the most noticeable properties of transition metal compounds is their characteristic colours. This colour is due to the presence of incomplete d-subshells or unpaired electrons, which result in the absorption of certain wavelengths of light and the reflection of others, giving the compound its characteristic colour. Transition metals and their compounds also act as good catalysts for various reactions. This is due to their unique properties, such as their ability to exhibit variable oxidation states, form unstable intermediate compounds, provide a large surface area for the adsorption of the reactant, and form dative bonds with ligands. In summary, the properties of transition metals make them important in many fields and are the reason for their widespread use in chemistry and engineering. Their unique properties, such as variable oxidation states, paramagnetism, and coloured compounds, make them ideal for a wide range of applications, including catalysis and materials science.
Properties of Transition Metals
Why do transition metals show magnetic properties?
Since most of the transition ions or their compounds have unpaired electrons in the d-subshell, this means that they are paramagnetic. In other words, they show magnetic properties.
What are five properties of transition metals?
1. High melting and boiling points2. Metallic character3. Strong4. High thermal conductivity5. High electrical conductivity
What are the characteristic properties of transition elements?
1. Complex ion formation2. Formation of coloured compounds3. Variable oxidation states4. Catalytic activity
Why do transition metals have different properties?
Transition metals have different properties due to the fact that they can lose electrons from the inner d-subshell, apart from the outer s-subshell. This means that they also have valence electrons in an inner shell, which is different from other elements which only have valence electrons in their outer shell.