Sliding Filament Theory
Sliding Filament Theory is the way muscles contract to make them strong. This theory tells us how muscles generate force.
Recap on skeletal muscle ultrastructure
Skeletal muscle cells have a long, tube-like shape and are called muscle fibres or myofibers for short. They have multiple nuclei because hundreds of smaller muscle cells combine during development. That's why they're called multinucleated cells. These muscles can grow to be quite big in humans.
Muscle fibre adaptations
Muscle fibres are specialised for contraction and have unique adaptations making them efficient. The plasma membrane of muscle fibres is called the sarcolemma, and the cytoplasm is called the sarcoplasm. Myofibers have a special smooth endoplasmic reticulum called the sarcoplasmic reticulum (), designed for storing, releasing, and reabsorbing calcium ions.
Inside myofibers, there are many contractile protein bundles called myofibrils. These myofibrils contain thick myosin and thin actin myofilaments, which are key proteins for muscle contraction. The arrangement of these proteins gives muscle fibres their striped appearance. It's important not to confuse myofibers with myofibrils.
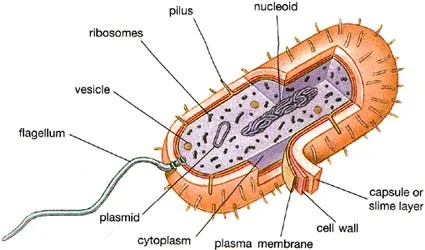
Skeletal muscle fibres also contain T tubules, which are extensions of the sarcoplasm into the centre of the myofibers (as seen in Figure 1). T tubules have a critical role in coupling muscle excitation with contraction, which is essential for muscle function. These fibres also contain many mitochondria, which are responsible for supplying the high amount of ATP required for muscle contraction. Additionally, having multiple nuclei allows muscle fibres to produce large amounts of proteins and enzymes required for muscle contraction.
Sarcomeres: bands, lines, and zones
The striated appearance of skeletal myofibers is due to the sequential arrangement and thin myofilaments in myofibrils. Each group of these myofilaments is called a sarcomere and is approximately 2 μm (micrometres) in length, with a cylindrical 3D arrangement. Z-lines, also known as Z-discs, border each sarcomere and are where the thin actin and myofilaments are attached.
In addition to actin and myosin, there are two other proteins found in sarcomeres that play a critical role in regulating the function of actin filaments in muscle contraction. These proteins are tropomyosin and troponin. During muscle relaxation, tropomyosin binds along actin filaments, blocking the actin-myosin interactions. Troponin is composed of three subunits: Troponin T, which binds to tropomyosin; Troponin I, which binds to actin filaments; and Troponin C, which binds to calcium ions.
Actin and its associated proteins form filaments that are thinner in size than the myosin, so they are referred to as the thin filament. In contrast, myosin strands are thicker due to their larger size and multiple heads that protrude outward, making them called thick filaments. The organisation of thick and thin filaments in sarcomeres gives rise to bands, lines, and zones within sarcomeres.

The sarcomere is split into the A and I bands, H zones, M lines, and Z discs. A band: Darker coloured band where thick myosin filaments and thin actin filaments overlap. I band: Lighter coloured band with no thick filaments, only thin actin filaments. H zone: Area at the centre of A bands with only myosin filaments. M line: Disc in the middle of the H zone that the myosin filaments are anchored to. Z-disc: Disc where the thin actin filaments are anchored to. The Z-disc Marks the border of adjacent sarcomeres.
The sliding filament theory
For a skeletal muscle to contract, its sarcomeres must shorten in length. The thick and thin filaments do not change; instead, they slide past one another, causing the sarcomere to shorten. This process is known as the sliding filament theory.
Evidence for the sliding filament theory
These changes occur due to the sliding filament theory, where the myosin heads of the thick filaments attach to the actin filaments and pull them towards the centre of the sarcomere. This process is powered by the hydrolysis of ATP, which provides the energy for the myosin heads to change shape and pull the actin filaments.
As the myosin heads continue to attach and detach from the actin filaments, the sarcomere shortens, causing the muscle to contract. This process is regulated by calcium ions, which bind to the regulatory proteins on the actin filaments, exposing the binding sites for the myosin heads. The sliding filament theory provides a mechanistic explanation for how muscles contract and generate force. By understanding the complex interactions between the myosin and actin filaments, researchers can develop new therapies and treatments for muscle-related disorders and injuries.

The sliding filament mechanism of muscle contraction
The process of muscle contraction and relaxation can be broken down into three main steps: muscle stimulation, muscle contraction, and muscle relaxation.
Muscle stimulation begins with an action potential arriving at the axon terminal of a presynaptic neuron, which triggers the release of acetylcholine into the synaptic cleft The acetylcholine binds to receptors on the muscle fiber, causing depolarization of the sarcolemma. The depolarization of the sarcolemma triggers the release of calcium ions from the sarcoplasmic reticulum, which bind to troponin C and cause a conformational change that leads to the movement of tropomyosin away from actin-binding sites. This allows for the interaction of actin and myosin filaments, resulting in the formation of cross-bridges. The energy released in the formation of cross-bridges powers a power stroke, which pulls the actin filaments towards the M line and causes the sarcomeres to shorten. This process continues as long as calcium ions are present in the sarcoplasm. Muscle relaxation occurs when the nerve impulse stops, and calcium ions are pumped back into the sarcoplasmic reticulum using ATP. This decrease in calcium ion concentration within the sarcoplasm causes tropomyosin to move and block the actin-binding sites, preventing any further cross-bridges from forming between actin and myosin filaments. This leads to muscle relaxation. Understanding the complex interactions between the nerve impulse, calcium ions, actin, and myosin filaments is essential for developing therapies and treatments for muscle-related disorders and injuries.

Source of energy for muscle contraction
Correct, energy in the form of ATP is essential for muscle contraction, and it can be generated through three different pathways: aerobic respiration, anaerobic respiration, and the regeneration of ATP using phosphocreatine. Aerobic respiration occurs in the mitochondria and involves the breakdown of glucose and the production of ATP through oxidative phosphorylation. Anaerobic respiration occurs in the cytoplasm and generates ATP through glycolysis, but without the use of oxygen. Finally, the regeneration of ATP using phosphocreatine involves the transfer of a phosphate group from phosphocreatine to ADP to generate ATP. All these processes provide the energy required for the sliding filament theory to produce muscle contraction. Understanding the different energy pathways that exist in muscles is essential for athletes, coaches, and trainers to optimize performance and prevent muscle fatigue and injury.
Sliding Filament Theory
How do muscles contract according to sliding filament theory?
According to the sliding filament theory, a myofiber contracts when myosin filaments pull actin filaments closer towards the M line and shorten sarcomeres within a fibre. When all the sarcomeres in a myofiber shorten, the myofiber contracts.
Does the sliding filament theory apply to cardiac muscle?
Yes, the sliding filament theory applies to striated muscles.
What is the sliding filament theory of muscle contraction?
The sliding filament theory explains the mechanism of muscle contraction based on actin and myosin filaments that slide past each other and cause sarcomere shortening. This translates to muscle contraction and muscle fibre shortening.
What are the sliding filament theory steps?
Step 1: Calcium ions are released from the sarcoplasmic reticulum into the sarcoplasm. Myosin head does not move. Step 2: Calcium ions cause tropomyosin to unblock actin-binding sites and permit cross bridges to form between actin filament and myosin head. Step 3: Myosin head utilises ATP to pull on actin filament toward the line. Step 4: Sliding of actin filaments past myosin strands results in shortening of sarcomeres. This translates to contraction of the muscle. Step 5: When calcium ions are removed from the sarcoplasm, tropomyosin moves back to block calcium-binding sites. Step 6: Cross bridges between actin and myosin are broken. Hence, the thin and thick filaments slide away from each other and the sarcomere returns to its original length.
How does sliding filament theory work together?
According to the sliding filament theory, myosin binds to actin. The myosin then alters its configuration using ATP, resulting in a power stroke that pulls on the actin filament and causes it to slide across the myosin filament towards the M line. This causes the sarcomeres to shorten.